Heat capacity of hydrogen sulfide at various temperatures. Specific heat capacity of natural gas
Not specific, but total thermal capacity, in the generally accepted physical sense, is the ability of a substance to heat up. At least that's what any textbook on thermal physics tells us - this is classic definition heat capacity(correct wording). In fact, this is an interesting physical feature. Little known to us in everyday life "side of the coin." It turns out that when heat is supplied from the outside (heating, warming up), not all substances react equally to heat ( thermal energy) and heat up differently. Ability GAS of natural origin used as fuel receive, receive, retain and accumulate (accumulate) thermal energy is called the heat capacity of NATURAL combustible gas. And herself heat capacity of methane, is a physical characteristic describing the thermophysical properties of gas fuel. At the same time, in different applied aspects, depending on a specific practical case, one thing may turn out to be important for us. For example: the ability of a substance to take warm or the ability to accumulate thermal energy or "talent" to keep it. However, despite some difference, in the physical sense, the properties we need will be described heat capacity of methane.
A small, but very "nasty snag" of a fundamental nature is that the ability to heat up - thermal capacity of methane, is directly related not only to chemical composition, the molecular structure of a substance, but also with its quantity (weight, mass, volume). Due to such an "unpleasant" connection, the general heat capacity of methane becomes too inconvenient physical characteristic of the substance. Since, one measured parameter simultaneously describes "two different things". Namely: really characterizes thermophysical properties of NATURAL GAS, however, "in passing" also takes into account its quantity. Forming a kind of integral characteristic, in which "high" thermal physics and a "banal" amount of matter (in our case: gas fuel) are automatically connected.
Well, why do we need such thermophysical characteristics, in which "inadequate psyche" is clearly traced? From a physics point of view, the total heat capacity of methane(in the most clumsy way), tries not only to describe the amount of thermal energy that can accumulate in gas fuel, but also "in passing inform us" about the amount NATURAL COMBUSTIBLE GAS. It turns out absurdity, and not a clear, understandable, stable, correct thermophysical characteristic of methane. Instead of a useful constant suitable for practical thermophysical calculations, we are given a floating parameter, which is the sum (integral) of the amount of heat received NATURAL GAS and its mass or volume of methane.
Thank you, of course, for such "enthusiasm", but the quantity NATURAL FUEL GAS I can measure myself. Having received results in much more convenient, "human" form. Quantity NATURAL COMBUSTIBLE GAS I would like not to "extract" by mathematical methods and calculations using a complex formula from the general heat capacity of methane, at different temperatures, but find out the weight (mass) in grams (g, g), kilograms (kg), tons (tons), cubes (cubic meters, cubic meters, m3), liters (l) or milliliters (ml). Especially since smart people long ago came up with measuring instruments quite suitable for these purposes. For example: scales or other devices.
Especially the "annoying floating nature" of the parameter: general heat capacity of naturally occurring GAS. His unstable, changeable "mood". When changing the "serving size or dose", heat capacity of NATURAL GAS at different temperatures changes immediately. More combustible gas mixture, physical quantity, absolute value heat capacity of methane- increases. Less amount of combustible gas mixture, value thermal capacity of methane decreases. "Disgrace" some turns out! In other words, what we "have" cannot in any way be considered a constant describing thermophysical characteristics of NATURAL GAS at different temperatures. And it is desirable for us to "have" an understandable, constant coefficient, a reference parameter that characterizes thermal properties gas fuel, without "references" to the amount of methane (weight, mass, volume). What to do?
This is where a very simple but "very scientific" method comes to our rescue. It comes down to not only bailiff "ud. - specific", before physical quantity, but to an elegant solution involving the exclusion of the amount of matter from consideration. Naturally, "uncomfortable, superfluous" parameters: mass or volume NATURAL COMBUSTIBLE GAS absolutely impossible to rule out. At least for the reason that if there is no amount of methane, then there will be no “subject of discussion” itself. And the substance should be. Therefore, we choose some conditional standard of mass or volume, which can be considered a unit suitable for determining the value of the coefficient "C" we need. For weight of NATURAL GAS fuel, such a unit of mass of methane, convenient in practical use, turned out to be 1 kilogram (kg).
Now we we heat one kilogram of NATURAL GAS by 1 degree, and the amount of heat (thermal energy) we need to heat gas mixture one degree - this is our correct physical parameter, coefficient "C", well, quite fully and clearly describing one of thermophysical properties of NATURAL GAS at different temperatures. Please note that now we are dealing with a characteristic describing physical property substance, but not trying to "additionally inform us" about its quantity. Comfortable? There are no words. It's a completely different matter. By the way, now we are not talking about the general thermal capacity of methane. Everything has changed. THIS IS THE SPECIFIC HEAT OF NATURAL GAS, which is sometimes called by another name. How? Just MASSIVE HEAT CAPACITY OF NATURAL COMBUSTIBLE GAS. Specific (ud.) And mass (m.) - in this case: synonyms, they mean here the one we need coefficient "C".
Table 1. Coefficient: specific heat capacity of NATURAL GAS (sp.). Mass thermal capacity of NATURAL combustible GAS. Reference data.
Simply put, heat capacity FROM body is called the amount of heat that needs to be expended in this process to heat the body by one degree: .
Usually, heat capacity is referred to a unit of the amount of a substance and, depending on the chosen unit, they distinguish:
specific mass heat capacity With, referred to 1 kg of gas, J/(kgK);
specific volumetric heat capacity With´, referred to the amount of gas contained in 1 m 3 of volume under normal physical conditions, J / (m 3 K);
specific molar heat capacity μ With, referred to one kilomole, J / (kmolK).
The relationship between the specific heat capacities is established by the obvious relationships:
With= μ With/μ; With´= Withρ n. (2.13)
Here ρ n is the gas density under normal conditions.
The change in body temperature with the same amount of heat supplied depends on the nature of the process taking place, therefore heat capacity is a function of the process. This means that the same working fluid, depending on the process, requires a different amount of heat for its heating by 1 K. Numerically magnitude With varies from + ∞ to – ∞.
In thermodynamic calculations great importance have:
heat capacity at constant pressure
With p = δ q p/ dT, (2.14)
q p , brought to the body in the process at constant pressure, to a change in body temperature dT;
heat capacity at constant volume
With v = δ q v /dT, (2.15)
equal to the ratio of the amount of heat δ q v, brought to the body in the process at a constant volume, to a change in body temperature dT.
In accordance with the first law of thermodynamics for closed systems δ q = du + pdv.
Taking into account the fact that (relations (2.1))
Δ q = (∂u/∂T) v dT + [(∂u/∂v) T + p]dv. (2.16)
For an isochoric process ( v=const) this equation takes the form , and, taking into account (2.15), we obtain that
c v = (∂u/∂T) v . (2.17)
For an ideal gas
(equation 2.2), so
c v = du/dT. (2.18)
For an isobaric process ( R=const) from equations (2.16) and (2.14) we obtain
c p = (∂ u/∂T) v + [(∂u/∂v) T + p](dv/dT) p
Since the internal energy ideal gas is determined only by its temperature and does not depend on the specific volume, then
.
(2.19)
From the Clapeyron equation (1.4) pv
= RT(1.3) follows , where
c p= With v + R. (2.20)
Relationship (2.20) is called Mayer's equation and is one of the main ones in technical thermodynamics of ideal gases.
In the process v\u003d const, the heat imparted to the gas goes only to change its internal energy, while in the process p \u003d const, the heat is spent both to increase the internal energy and to perform the work of expansion. That's why c p more With v by the amount of this work.
Formula determines the so-called true heat capacity, i.e., the value of the heat capacity at a given temperature. In practice, heat capacity is widely used to calculate the amount of heat q 12 , which must be spent to heat the body from one temperature ( t 1) to another ( t 2) (or which will be released upon cooling from t 2 to t 1).
If the heat capacity does not change in the indicated temperature range, then
, kJ/kg and
, kJ. (2.21)
This formula can also be used for approximate (not very accurate) calculations. For example, the specific heat capacity of water at room temperature is 4.187 kJ/(kgC), and air 1 kJ/(kgC). Since the heat capacity of almost all substances changes (most often increases) with increasing temperature, it is necessary, strictly speaking, to use the formula
.
(2.21a)
On fig. 2.2 magnitude q 12 is shown as a shaded area. It would seem that the easiest way, by applying the mean value theorem, is to write
,
(2.22)
where is the average heat capacity in the temperature range t 1 …t 2 .
Rice. 2.2. Dependence of true heat capacity on temperature
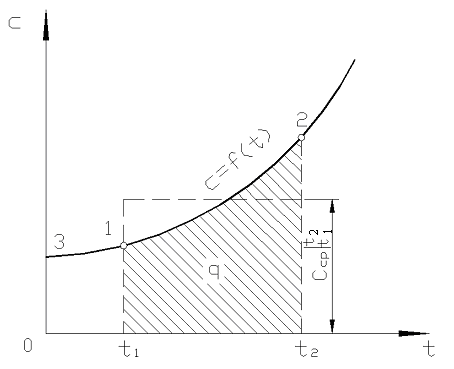
However, for real calculations, such a notation is very inconvenient, since the heat capacity is chosen from tables, and depends on how t 1 and from t 2. A function table of two arguments would be very cumbersome.
In practice, proceed as follows. The desired amount of heat is presented as the difference between its amounts required to heat the body from 0 С to temperatures t 2 and t 1 respectively (Fig. 2.2)
. (2.23)
,
(2.24)
where - average heat capacity in the temperature range 0… tC.
The value of the average heat capacity for a given substance (and a given process, i.e. With R or c v) depends only on one temperature t. It is these heat capacities that are given in all tables under the name "average". What has been said is true for With
(With R and c v), as well as for With and With. As an example, the table below shows the heat capacity versus temperature for some of the most common gases. It should be emphasized that in formula (2.24) the temperature is substituted in degrees Celsius.
The average molar heat capacity of various gases at R=const
(according to M.P. Vukalovich, V.A. Kirillin, V.N. Timofeev)
t, C |
With R, kJ/(kmolK) |
|||||||
N 2 atmospheric | ||||||||
In this table, the values With p are given through 100 С. Since they do not change much with temperature, intermediate values can be found by linear extrapolation. Recall how this is done. There are tabular function values y
= f(x) at 1 (when X= X 1) and at 2 (when X= X 2). Function value at(X) at X 1 <X<X 2 equals . This formula is true both for a monotonically increasing function (when at 2 >at 1) and for monotonically decreasing ( at 2 <at one); In this case, the fraction will be negative, since X 2 > X 1 always.
The table shows the values of thermal conductivity of gases depending on temperature and pressure.
Thermal conductivity values are indicated for temperatures in the range from 20 K (-253 °C) to 1500 K (1227 °C) and pressure from 1 to 1000 atmospheres.
Table given the thermal conductivity of the following gases: , , freon-14 CF 4 , ethylene C 2 H 4 . The unit of thermal conductivity is W/(m deg).
It should be noted that the thermal conductivity of gases increases with increasing temperature and pressure. For example, the thermal conductivity of ammonia gas at room temperature and normal atmospheric pressure is 0.024 W / (m deg), and when it is heated by 300 degrees, the thermal conductivity increases to a value of 0.067 W / (m deg). If the pressure of this gas is increased to 300 atmospheres, then the thermal conductivity value will become even higher and will have a value of 0.108 W / (m deg).
Note: Be careful! The thermal conductivity in the table is indicated with a factor of 10 3 . Don't forget to divide by 1000!
Thermal conductivity of inorganic gases depending on temperature
The table gives the values of the thermal conductivity of inorganic gases depending on the temperature at normal atmospheric pressure. The values of thermal conductivity of gases are indicated at temperatures from 80 to 1500 K (-193 ... 1227 ° C).
The table shows the thermal conductivity of the following gases: nitrous oxide N 2 O, sulfur hexafluoride SF 6, nitric oxide NO, hydrogen sulfide H 2 S, ammonia NH 3, sulfur dioxide SO 2, steam H 2 O, carbon dioxide CO 2, heavy water steam D 2 O, air.
It should be noted that the thermal conductivity of inorganic gases increases with increasing gas temperature.
Note: The thermal conductivity of gases in the table is indicated with a factor of 10 3 . Don't forget to divide by 1000!
Thermal conductivity of organic gases as a function of temperature
The table shows the thermal conductivity values of organic gases and vapors of some liquids depending on temperature at normal atmospheric pressure. The values of the thermal conductivity of gases are given in the table in the temperature range from 120 to 800 K.
The thermal conductivity of the following organic gases and liquids is given: acetone CH 3 COCH 3, octane C 8 H 18, pentane C 5 H 12, butane C 4 H 10, hexane C 6 H 14, propylene C 3 H 6, heptane C 7 H 16, amyl alcohol C 5 H 11 OH, xylene C 8 H 10, isopropyl alcohol C 2 H 7 OH, methane CH 4, methyl alcohol CH 3 OH, carbon tetrachloride CCl 4, cyclohexane C 6 H 12, ethane C 2 H 6, carbon tetrafluoride CF 4, freon-11 CFCl 3, ethyl chloride C 2 H 5 Cl, freon-12 CF 2 Cl 2, ethylene C 2 H 4, freon-13 CF 3 Cl, ethyl formate HCOOC 2 H 5, freon-21 CHFCl 2, diethyl ether (C 2 H 5 ) 2O.
As can be seen from the table, the value of thermal conductivity of organic gases also increases with increasing gas temperature.
Be careful! The thermal conductivity in the table is indicated with a factor of 10 3 . Don't forget to divide by 1000! For example, the thermal conductivity of acetone vapor at a temperature of 400 K (127°C) is 0.0204 W/(m deg).